A Comprehensive Guide to Protein NMR Spectroscopy
Written on
Chapter 1: Understanding Protein NMR Spectroscopy
Nuclear Magnetic Resonance (NMR) spectroscopy is a distinctive method among atomic-resolution techniques used to analyze the structures of biological macromolecules. One of its standout features is its capability to function in a solution state at nearly room temperature. By utilizing the magnetic characteristics of certain atomic nuclei, NMR spectroscopy can examine the structure, dynamics, and interactions of biological macromolecules with atomic precision.
Initially, a sample is positioned within a robust static magnetic field, often several Tesla in intensity, aligning the magnetic spins of active nuclei such as ¹H, ¹³C, ¹?N, and ³¹P—elements crucial to biological research. These nuclei are recognized as NMR-active due to their net magnetic moments that respond to the applied magnetic field. While ¹H and ³¹P are naturally abundant and easily identifiable, ¹³C and ¹?N are less prevalent, frequently necessitating isotopic enrichment for significant data collection, particularly in protein studies.
Following the alignment within the magnetic field, one or more radiofrequency pulses are administered to disturb the magnetization of the nuclei, utilizing channels specifically aimed at each isotope. A meticulously crafted sequence of pulses yields an NMR signal that encompasses various types of information. By the conclusion of the pulse sequence, the magnetization typically shifts to the ¹H channel, where it is recorded over time. A Fourier transform of this time-dependent signal results in a frequency-dependent representation known as the NMR spectrum. Through the generation of “indirect” signals, these pulse sequences can produce not only one-dimensional spectra but also 2D, 3D, and even higher-dimensional spectra correlating signals from different nuclei. Notably, the ¹H,¹?N-Heteronuclear Single Quantum Coherence (HSQC) spectrum plays a vital role in protein NMR, as will be elaborated later in this introduction.
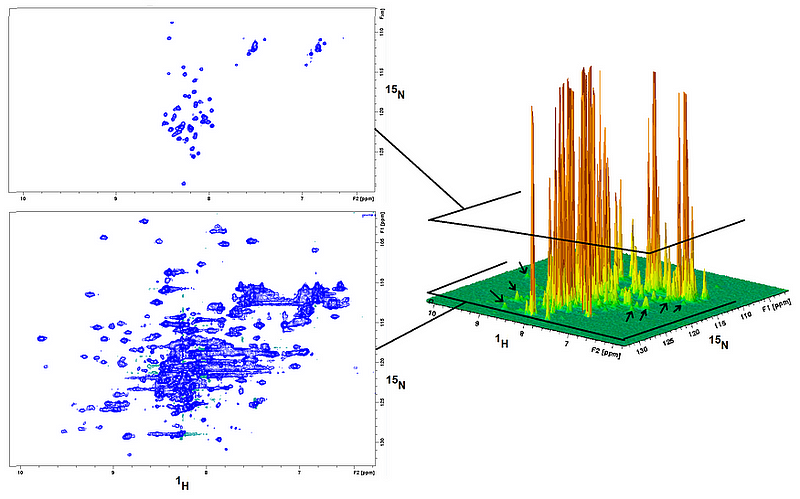
Section 1.1: Insights from NMR Spectra
The resonances detected in an NMR spectrum convey rich, indirect information regarding the atom that produced them, its immediate surroundings, the molecule it belongs to, and the solvent in the vicinity. The position of a resonance in the spectrum, known as the chemical shift, indicates the electronic and magnetic environment of the nucleus. Scalar and dipolar couplings arise when nuclei are sufficiently close to exchange magnetization through various mechanisms, providing insights into covalent connections and spatial arrangements among atoms. The resonance linewidth—its width at half height—reflects the dynamics and chemical exchange processes experienced by the atom, including movement, solvent exchange, and transient interactions with other atoms.
Section 1.2: Beyond Static Structures
These characteristics render NMR an invaluable tool for comprehending not only the static structures of biomolecules but also their dynamic behaviors and interactions in solution, under conditions that closely approximate their natural environments. By working in solution and at near-physiological temperatures, NMR enables the examination of structural and various biophysical properties of proteins and other biological macromolecules in conditions akin to those they face in their natural states—especially when compared to the crystalline states employed in X-ray diffraction experiments or the cryogenic conditions used in Cryo-EM. Specialized techniques even facilitate the exploration of biological macromolecules within living cells using solution NMR.
Importantly, NMR differentiates itself from other atomic-resolution methodologies not only through its capability to resolve molecular structures—although it does have limitations in this aspect due to factors like protein size—but also in its ability to investigate folding, aggregation, dynamics, intermolecular interactions, and various other properties and mechanisms. It is particularly adept at studying interactions among multiple molecules, being able to detect even weak reversible binding in the millimolar range. NMR can yield structural information about intermolecular complexes without requiring complete structure determination, unlike Cryo-EM or X-ray crystallography, which necessitate resolving the 3D structure of a stable complex to obtain such information.
Chapter 2: The ¹H-¹?N HSQC Experiment
Central to these investigations is the ¹H-¹?N HSQC experiment, a 2D technique that correlates nitrogen atoms with their directly bonded hydrogen counterparts. This rapid and sensitive experiment typically serves as a fingerprint to ascertain whether a ¹?N-labeled protein sample is folded as anticipated. The strength of the ¹H-¹?N HSQC spectrum lies in the fact that each “crosspeak” corresponds to a specific backbone unit, enabling researchers to monitor shifts in position and intensity during titration, pH alterations, solvent exchanges, temperature variations, and other conditions.
For instance, during the titration of an unlabeled molecule into a ¹?N-labeled protein sample, one might observe peak shifts signifying weak and rapid exchanges between free and bound forms, intensity variations indicating stronger or slower interactions, or no change if there is no interaction. By mapping these effects onto the protein’s sequence or structure, researchers can extract detailed information about the interaction and potentially dock molecules to create a low-resolution, experiment-supported model of the complex.
Chapter 3: Challenges in NMR Spectroscopy
Despite its numerous advantages, NMR spectroscopy does face limitations. Solution-state NMR is often difficult to apply to proteins exceeding a few tens of kilodaltons, and even for smaller proteins, it presents specific buffer requirements while demanding relatively large quantities of protein—similar to what is needed for X-ray diffraction, but significantly more than what is required for Cryo-EM. Moreover, many NMR experiments require protein samples enriched in ¹?N and/or ¹³C isotopes, which are NMR-active alongside ¹H. This enrichment is easily achievable for proteins that can be produced recombinantly in E. coli cells but is neither routine nor cost-effective for proteins that need to be expressed in different cell types. Additionally, reagents for ¹³C enrichment can be quite expensive.
Another significant challenge is NMR’s inherently low sensitivity, which necessitates concentrating protein samples to several hundred micromolar and ensuring their stability at the measurement temperature for extended periods, often spanning hours to days. Achieving high protein solubility and stability may require engineering soluble mutants of the target protein. Buffer conditions also impose constraints, as ideal solutions should be buffered between pH 4 and 7 with minimal salt, though some proteins might require high salt concentrations. Depending on the NMR probe, there may be a limit around 250 mM NaCl. Thus, a careful balance must be maintained between obtaining high-quality spectra and ensuring protein stability.
Series: The Power of NMR Spectroscopy in Biological Research
As I continue writing the subsequent chapters, here are the links to Chapters 1 through 3:
- Nuclear Magnetic Resonance Spectroscopy: The Overlooked Powerhouse of Biology - Chapter 1
- Nuclear Magnetic Resonance Spectroscopy: The Overlooked Powerhouse of Biology - Chapter 2
- Nuclear Magnetic Resonance Spectroscopy: The Overlooked Powerhouse of Biology - Chapter 3
For more insights and updates, you can visit my website: www.lucianoabriata.com. I write about various topics that intrigue me, including nature, science, technology, and programming. Subscribe for the latest updates, or reach out to consult about small projects. Feel free to tip me if you find my work valuable.